
Efficient, Scalable, Evolutionary: Lab Design Strategies Inspired by the Respiratory System
Future Labs is HDR’s series of design strategies that viably and economically address the environmental challenges that complex lab buildings create, drawing on inspiration from human, plant and animal bodies and symbiotic relationships in the natural world.
Historically, higher air change rates in biomedical laboratories have been thought to keep laboratorians and others sharing space in research buildings safe from hazards due to poorly performing primary containment, fugitive chemicals released into the laboratory air and the risk of chemical spills. At the same time, these systems have encompassed one of the most energy-intensive aspects of lab buildings, though systems must allow for variances regarding location and climate.
We challenged this conventional lab design and engineering approach to ventilation as part of Future Labs. The coevolution of science, safety and building technology gives us an opportunity to reevaluate the fundamental assumptions behind how and why we design based on ventilation systems — the lungs of the lab.
We asked, "Can air change rates based upon zoning activities within a changing and evolving lab paradigm be more energy efficient without sacrificing or even enhancing safety?"
The Lungs of the Lab
From physical exertion to sleep, our metabolic state determines the pace of our respiratory system, supplying just the right amount of air we need at any given moment. Air entering our bodies is filtered and conditioned while traveling downstream through a network of ever-shrinking channels described by a property called Murray’s Law. In a wonder of evolutionary engineering, molecules of nitrogen, oxygen and trace gases travel further and further into the network until they reach one of roughly 300 million air sacs in the lungs, providing enough surface area for effective gas transfer to the blood in a package that fits snugly inside our chest. The respiratory system conditions and filters air, preparing it for use in our lungs.
When we look at laboratories, the mechanical systems moving air through our buildings resemble these pathways. And just as our lungs are an important part of the body’s system to remove contaminants and waste, ventilation air in a lab building carries away contaminants introduced by lab processes. However, labs have not historically been designed to adapt to the "metabolic rate" of lab activity.
We asked, "Can labs be designed to efficiently respond with appropriate air change rates for differing levels of hazard and spill management practices?"
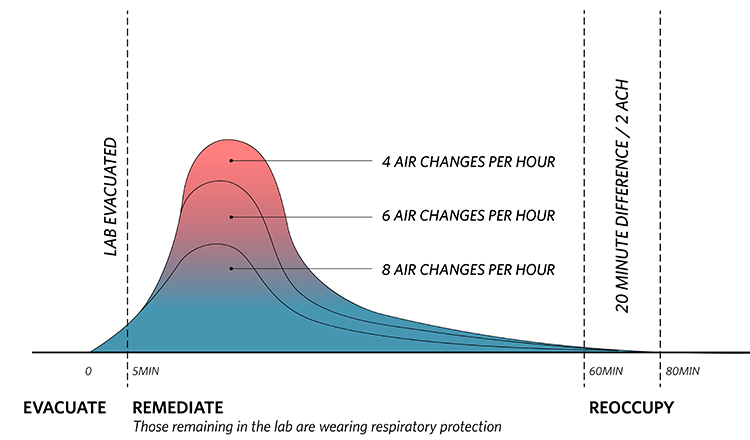
Inhale: How Much Air Is Needed in the Lab?
A 2009 study from Yale University gives us some insight into some of the efficiencies that can be gained by re-evaluating standard protocols. In the study, the researchers modeled what happens to the chemical concentration in lab air after a spill or point source chemical emission. Specifically, they looked at how air change rates (the number of times the room’s air is replaced with fresh air) of 4 air changes per hour (ACH), 6 ACH or 8 ACH at the time of the spill. At first glance, the fact that the contaminant concentration is much higher within 20 minutes of a spill at 4 ACH as compared to 6 ACH and 8 ACH seems to support providing higher ventilation and air change rates in labs, as developed and implemented in the 1970s.
This is where it is important to assess how policies and Environmental Health & Safety (EHS) guidelines overlap with ventilation requirements. Depending on the chemical spilled and the exposed population, it is entirely likely that none of the concentrations shown would be deemed safe by an industrial hygienist or EHS team in a modern lab. Conversely, it might be deemed that all are safe depending on the specific chemical spill. Given all the factors involved in making an immediate determination of the safety of staying in the lab, the lab space would be evacuated, and the spill would be addressed by trained personnel in appropriate personnel protective equipment. Occupants would not return until the lab had been deemed safe for the occupants to resume their work.
In either case, lab personnel would be forced out of the lab for some period of time regardless of the air change rate and there is actually a small difference, in this case, about 20 minutes, in the loss of use of the lab between 4 ACH and 8 ACH.
Exhale: How Often Do Labs Reach A Problematic Level of Contamination?
Furthermore, research shows that contamination requiring ventilation responses in typical research labs is rare. A study included in the International Institute for Sustainable Laboratories’ (I2SL) Best Practices Guide series surveyed roughly 1.5 million hours of operating data from facilities equipped with the Aircuity demand control ventilation (DCV) management system and found that detected total volatile organic compounds (TVOC), representing a wide range of common laboratory reagents, remained below commonly accepted safe levels over 99% of the hours studied. This ratio, applied to a single hypothetical facility over a year, would result in 70 hours of increased ventilation demand per year. Most of the time with detected TVOCs above the maximum safe level were slightly above, only requiring a modest increase in ventilation rates.
This scenario begs the question of whether it makes sense to operate a lab at high ventilation rates at all hours over the decades-long lifespan of the lab, in exchange for the 20 extra minutes of work time after chemical spills which are exceedingly rare. We need an approach that is more responsive to the activity occurring within the labs.
Risk-Based Zoning: A Metabolic Lab Design Strategy
Historically, most labs were set up to handle the most hazardous conditions and, therefore, were all exposed to the same risk of chemical spill. A first step towards more “responsive” systems for labs is risk-based zoning. In today’s labs, some spaces produce more, or more dangerous, contaminants than others, requiring high ventilation rates. However, like the previously referenced I2SL study, many lab spaces produce relatively few or lower-risk contaminants. Recognizing this, a standard but oft-overlooked strategy of lab design and operation is risk-based zoning, where risks due to lab processes are physically separated and stratified. As part of this strategy, after hazards and risks are identified, everything from ventilation rates to access control to personal protective equipment requirements are selected to match the assessed risk. Ideally, but still too rarely, these risk mitigation strategies are based in part on a comprehensive lab ventilation risk assessment which results in a lab ventilation management plan.
How Does Risk-Based Zoning Work?
When neighboring zones have different risk levels, air pressure relationships provide containment to the higher-risk zone, which is kept at a negative pressure relative to the lower-risk zone to create directional airflow. In the past, this standard configuration was accomplished by designing constant volume air flow rates with increasing offsets between supply and exhaust corresponding to higher hazards. Simply put, air from the lower-risk zone moved to the higher-risk zone because the higher-risk zone is removing more air than is directly supplied to it, establishing and sustaining directional airflow. This effect can be stacked across multiple zones, in what is commonly called an air flow cascade.
A natural and unavoidable side effect of this pressure relationship is the transfer of air from a higher-pressure zone to any connected lower pressure zone allowing air to be used for ventilation in one zone and then transferred to the next as ventilation or makeup air, and even to another zone beyond that. Transferring air means that each zone’s ventilation air requirement (fresh air going into a space) can be less dependent on its exhaust requirement (contaminated air being removed from the building).
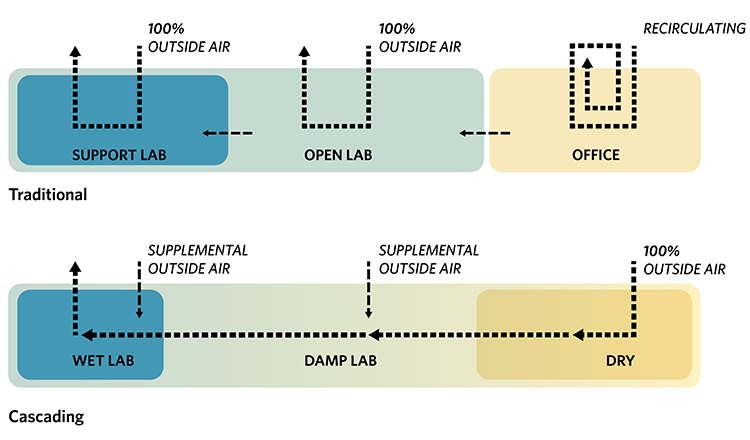
In biomedical labs, three basic zones are emerging with respect to the interaction between hazards and engineering controls. These zones result from the natural workflow of these labs, where working quantities of hazards are generally lower than in the past, analytical instrumentation is ubiquitous and lab environments offer a variety of functional space typologies to meet the needs of research.
- "Wet" Lab Zone: The "wet" zone is the highest risk zone where the majority of higher-risk chemicals, biological hazards and radioisotopes are handled. Ventilation in this zone is determined by exposure control devices appropriate to the hazard. This zone maintains a constant negative pressurization relative to surrounding spaces and is characterized by the presence of fume hoods, biosafety cabinets and the constant hum of moving air they create. Keeping major chemical paths within this zone limits the movement of chemicals within other areas of the facility, reducing the chances of spills and increasing safety.
- "Damp" Lab Zone: The "damp" zone is what most people think of as the typical open laboratory — people working in lab coats at benches, using buffers and other relatively innocuous chemicals in processes that are safe on the bench. In the “damp” open lab zone, hazards can be engineered out of the environment through closed systems or controlled through point source exhaust. Opportunities for lower ventilation rates should be explored here. This zone is positively pressurized to the wet lab and negatively pressurized to the dry lab.
- “Dry” Research Zone: The “dry” research zone is potentially comprised of two areas — dry "laboratory" space where analytical equipment is used with negligible chemical or biological hazards, and dry office, or “write-up", space where computing and analytical work is done. Ventilation in both cases should be similar to office areas, or as low as the Authority Having Jurisdiction, known as AHJ, or resident industrial hygienist allows in the dry laboratory space. This zone is positive to damp or wet laboratory spaces. Any sample preparation required prior to analysis has already been conducted in the wet or damp lab zone, reducing chemical use in the dry laboratories to small quantities of a few basic solvents or cleaners.
Risk-based mitigation airflow strategies result in:
- Cumulative air change of 1.5 ACH
- Approximate 34 percent reduction for heating/cooling/ventilation energy use intensity
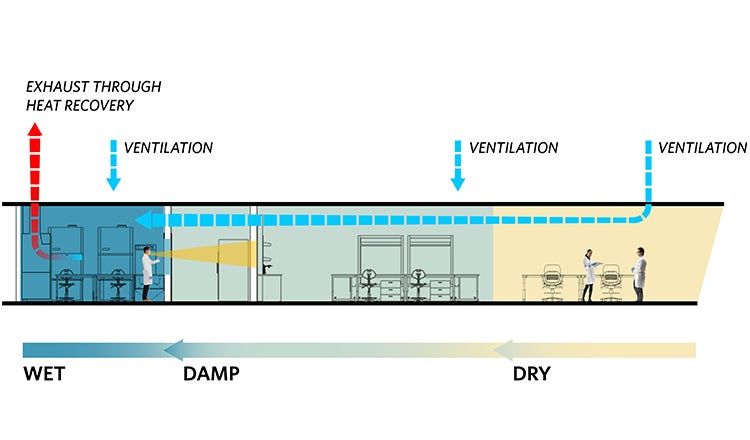
To date, the evolution towards risk-based zoning has been organic, borne of purely functional considerations. The fact that these three types of labs can also serve as a framework for reducing overall air changes is a useful side effect of the basic functionalities needed in modern labs. Researchers want to keep noise and hazards away when they are focused on analysis, and they want to keep their expensive instrumentation away from potential chemical spills — naturally wanting to separate dry zones from wet zones. Building owners and operators want to maximize space utilization and efficiency, while reducing long-term ownership costs and expensive renovations — making it advantageous for them to dedicate different zones based on the infrastructure required for operations. These trends paired with tailored lab ventilation management plans, codes and standards that allow for non-prescriptive design paths and modern air flow controls systems present opportunities for deep efficiency gains in laboratory facility mechanical systems.
Future Opportunities
Following the biomorphic analogy, where the body self-regulates respiration according to activity, labs should self-regulate their ventilation according to detected activity in the lab. When people sleep, breathing is minimized; when labs are unoccupied, they should provide minimum ventilation; when people exert themselves, they breathe heavily; when labs are in heavy use, mechanical systems need to respond at designed capacity. Fundamentally, oxygenated blood circulates to all parts of the body, leaving precisely the right amount of oxygen at each point along its route. Similarly, ventilation air should be circulated through the mechanical system to the points where it is needed, only at the rate required, even using some volume of air to serve multiple spaces in a single pass when possible.