
What Nanoscience Can Teach Us About Molecular Engineering Facilities
Ever since the noted Massachusetts Institute of Technology scholar Arthur von Hippel published the 1956 article "Molecular Engineering" in the journal Science, it has grown as a strategic approach to problem-solving. Molecular Engineering is a bottom-up approach that builds materials — both organic and inorganic — from atoms and molecules to meet the challenge at hand. One ubiquitous example is the development of organic light emitting diodes or OLED's, in use in smart phones. Immunotherapy examples include customized antibodies such as Abciximab for thrombosis. Much like the application of nanotechnology that came before, molecular engineering is not a separate engineering or scientific discipline but rather a methodology that crosses many different fields.
As evidence of molecular engineering’s growing relevance, the University of Chicago's Pritzker School of Molecular Engineering has recently been joined by the University of Washington's Molecular Engineering and Sciences Institute and Kyoto University in offering doctorates in this growing field. Additionally, the National Science Foundation has approved grants applying molecular engineering approaches to research as diverse as artificial intelligence, renewable energy, biophysics, semiconductor substrates, and reproducible cells.The NIH currently lists over 1900 grants for fiscal 2021 directly or indirectly applying the principles of molecular engineering.
Research institutes and universities must now consider how they will meet the growing facility demands for molecular engineering to support and enhance this emerging approach. In molecular engineering today, there are aspects of mechanical engineering, materials science, bioengineering, electrical engineering, solid-state and quantum physics, and synthetic biology. A facility must accommodate the critical mass of talent among classical disciplines to span the spectrum from molecular design theory to material production and system design to product development.
We can look to the interdisciplinary medical research buildings that emerged during the 1960s and nanoscience research facilities between the 1980s to 2000s to provide insight into molecular engineering facility design, which requires similar building types and strategies.
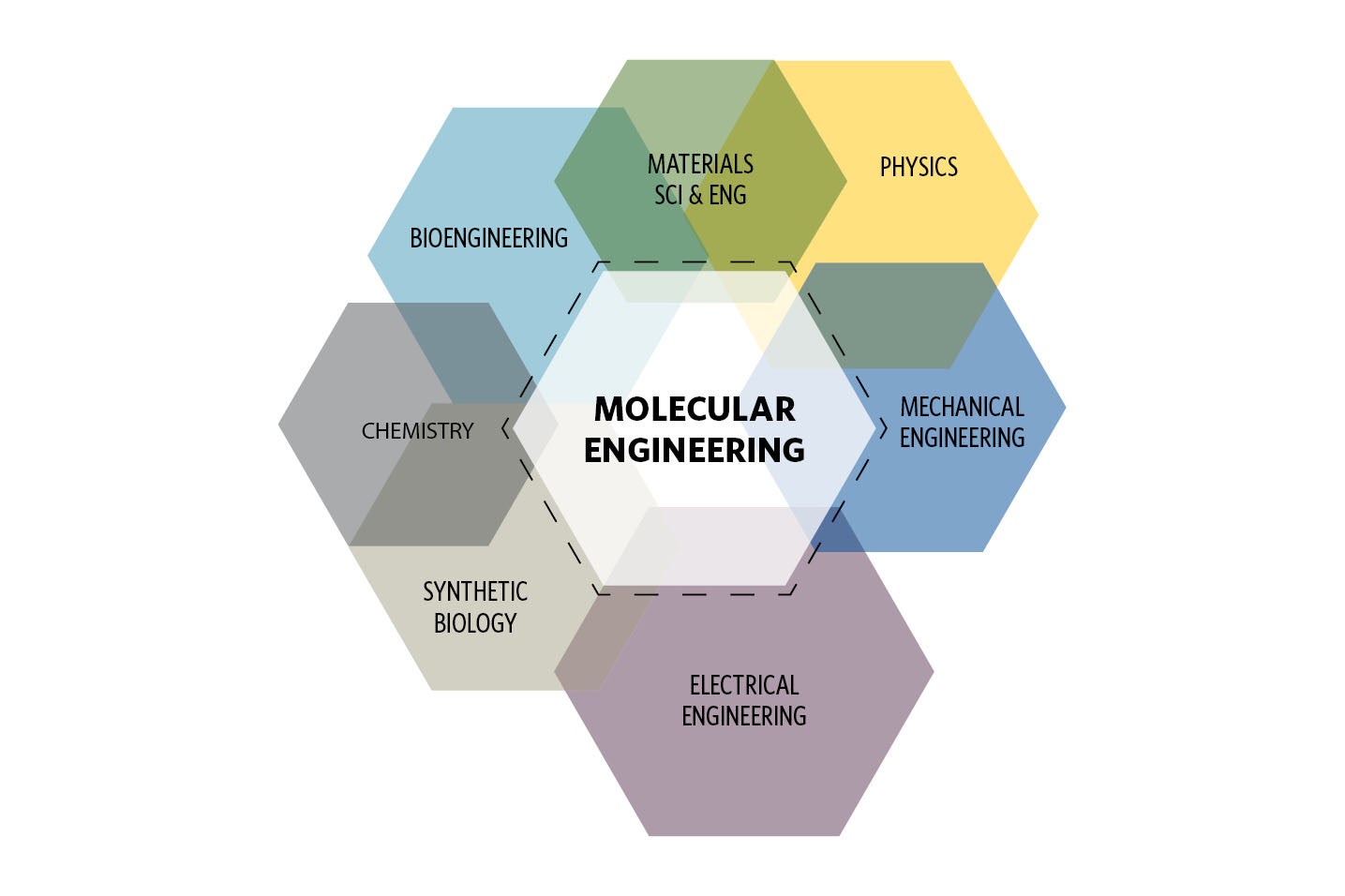
1. Model an Institute, Not a Department
Similar to most nanoscience research facilities, molecular engineering facilities should be operated in a way that forgoes concentration on a traditional department or discipline. Molecular engineering research is often multidisciplinary, and quite often the building will be managed by an institute or research centre. By keeping the building departmentally agnostic, the occupants and research can then be thematic or problem-focused. This is extremely helpful in removing the department-centric focus on a traditional engineering discipline that can lead to territorialism and inhibit critical interdisciplinary collaboration.
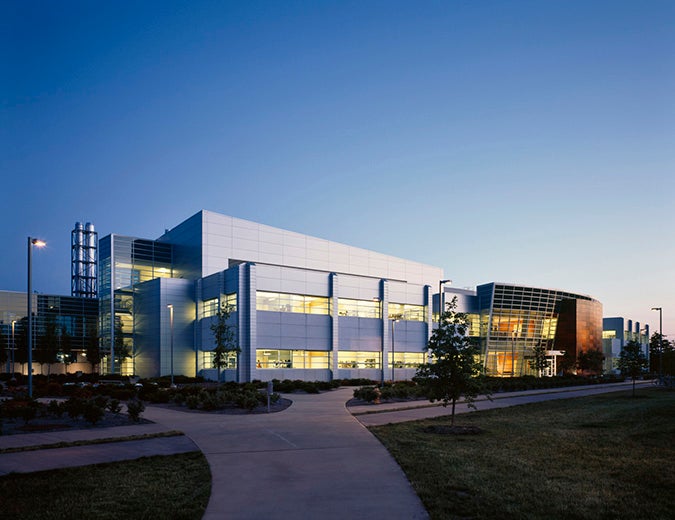
2. Think of the Facility as a “Kit of Tools”
Since molecular engineering is transdisciplinary in nature it requires processes, investigative techniques, instruments, and materials that are not always compatible. Therefore, its important to offer a wide variety of “tools” within the facility. Again, nanoscience research buildings can serve as a guide, as they faced similar challenges. Successful nanoscience and molecular engineering research facilities will both house varied toolsets, from chemical and material labs to computational space to advanced imaging and cleanrooms. They are highly collaborative spaces and make provisions for both casual and formal interaction among engineers and scientists. They offer relief from long periods of study or work.
Some of these tools call for precision-controlled environments and these spaces will be purpose built. Examples of these types of core labs include catalysis research involving high-pressure hazardous gases, advanced imaging labs housing electron microscopes of many types, cleanrooms for biological use or materials research, and labs for analytical tools like nanoscale secondary ion mass spectrometry or nuclear magnetic resonance spectroscopy.
3. Design for Adaptability
The design of molecular engineering facilities should be adaptable as techniques and processes evolve. While these facilities must include some purpose-built core labs as noted above, they must also have easily modified lab space to be successful. As the field of molecular engineering evolves, the needs of the research will inevitably change, and the building supporting them must adapt accordingly.
Conclusion
The world today is reaping the rewards of the nanotechnology boom of 30 years ago when emerging techniques enabled investigations at an incredibly small scale. The National Nanoscience Initiative provided support for these applications and helped to prompt a demand for unique facilities capable of supporting them in an interdisciplinary setting. In much the same way, molecular engineering represents an intellectual revolution provoking a need for multi-capability buildings offering a kit of diverse tools. Fortunately, we can look to the nanotechnology wave to provide a guideline for nascent molecular engineering facility design models.